The not-so-permanent frost
Rapid thawing of the Himalayan ice-cap is compounded by little-studied melting of permafrost that destabilises peaksA warming atmosphere also warms perennially frozen mountains. The stability of frozen rocks decreases with increasing temperature similar to how butter just taken out of a fridge becomes softer and less resistant to the cutting force of the knife.
Thawing frozen mountains means softening them, making them less resistant against gravity, leading to breakage of small to large sections, resulting in rock falls, rock avalanches, and landslides.
There is growing concern about the impact of global warming on the stability of icy peaks in mountain ranges around the world, which several recent events in the Himalaya well illustrate.
Permafrost is a technical short form of ‘permanent frost’ for frozen ground which remains at temperatures below zero degrees throughout the year.
Permafrost is below the surface, is not directly visible, and therefore often escapes the attention of scientists and political authorities. Sophisticated methods must be used to explore it, such as drilling, geophysical soundings, or climate-related modelling.
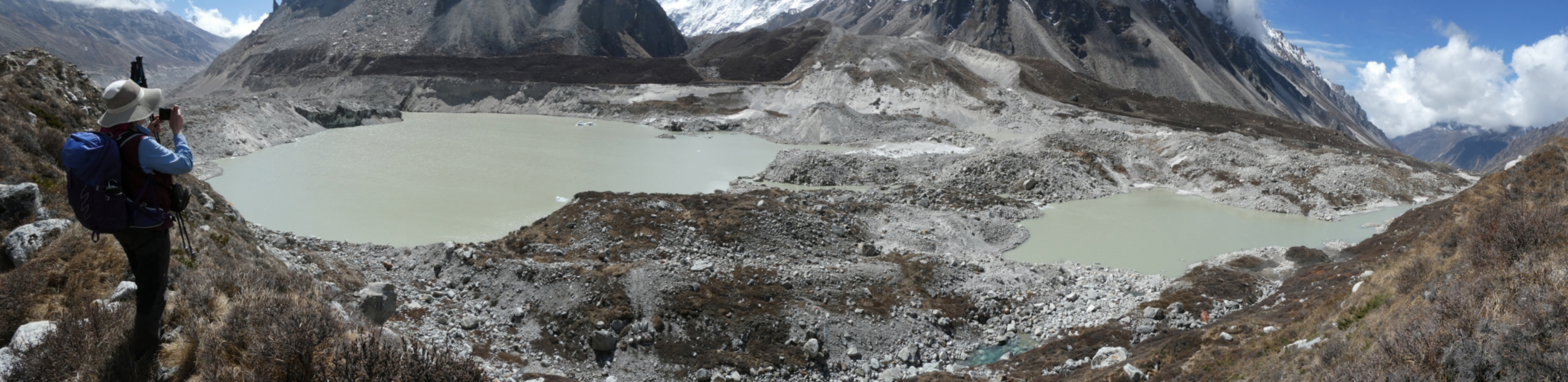
Generally, temperatures at surface level and directly below match the temperature of the air immediately above it. Polewards to around 60°N and S latitude, mean annual air and ground temperatures are mostly below 0°C. Permafrost is widespread in Alaska, northern Canada and Siberia.
The lower limit of negative mean annual temperatures is at 2,000-3,000m at mid-latitudes such as the European Alps or the Southern Alps of New Zealand, to 3,000-4,000m in the Himalaya, and to 5,000m near the equator in the Peruvian Cordilleras.
Above these elevations, permafrost affects peaks. In high mountains the permafrost area is often far larger than their glacier-covered regions. In Nepal, the permafrost-affected area is about 2 to 3 times bigger than the glacier area, while in China with the perennially frozen Tibetan Plateau this factor is about 30.
Temperatures decrease by 6-7°C for every 1,000m of altitude increase, and the coldest and most deeply frozen rocks are found in the highest parts of the peaks. The frozen zone inside mountains can reach depths of hundreds of metres. It is not just the ice on peaks in the European Alps or the Himalaya that is frozen throughout the year, but so are the rocks.
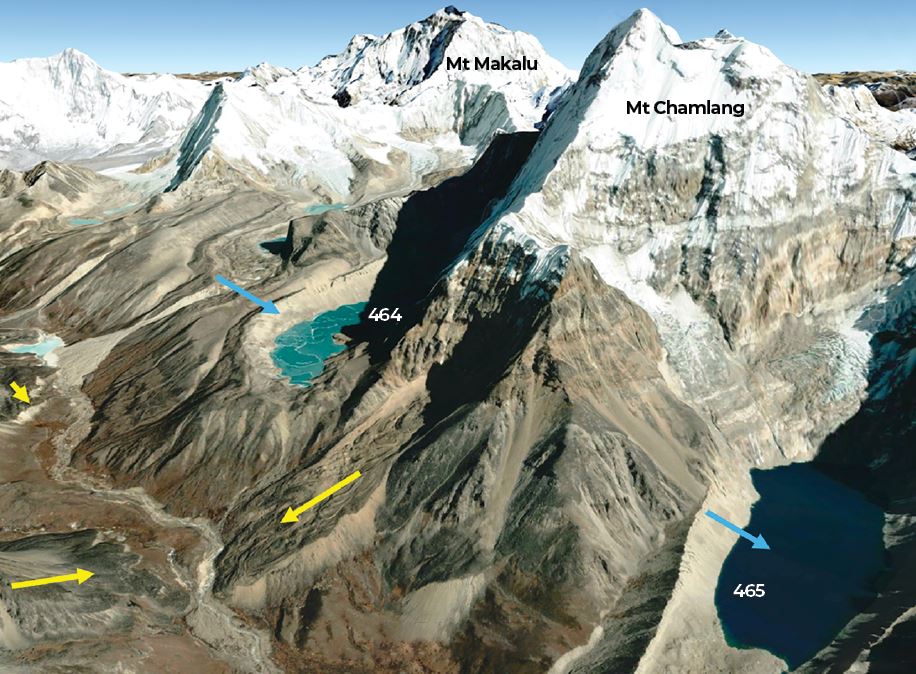
The sunny side of mountains are warmer, and the permafrost is thinner there than slopes in mountain shadow. Temperatures are not constant throughout the year, so a thin surface layer up to a few metres on top of permafrost that thaws in summer and freezes in winter. This seasonal freezing and thawing surface layer on permafrost is called the ‘active layer’.
Deep, long-term freezing influences geomorphic processes inside mountains, drastically changing their properties. Growth of ice in cracks and fissures within solid rock tends to expand already existing openings, and even create new ones. The related weakening of bedrock, however, is compensated by strong adhesion and increasing strength induced by the ice fillings and related cold temperatures.
Read also: Tracing past glacial floods in Kangchenjunga, Alton C Byers
Not only the ice itself, but also rocks, rock-ice, and rock-rock contacts increase their strength with lower temperatures. Perhaps most important is the effect of freezing and ice filling of cracks between rocks containing water. Deep frozen rocks are essentially impermeable to water as an often-decisive factor of stability inside mountain slopes.
In the abundant debris masses at the foot of high mountains, deep, long-term freezing causes even more spectacular changes. Formation of ice inside and beyond the pores can create a mixture of ice and debris with ice lenses, enabling such ice-rich frozen masses to slowly creep down the slopes of mountains. Landforms resulting from permafrost creep over past millennia look like lava streams and are called ‘rock glaciers’.
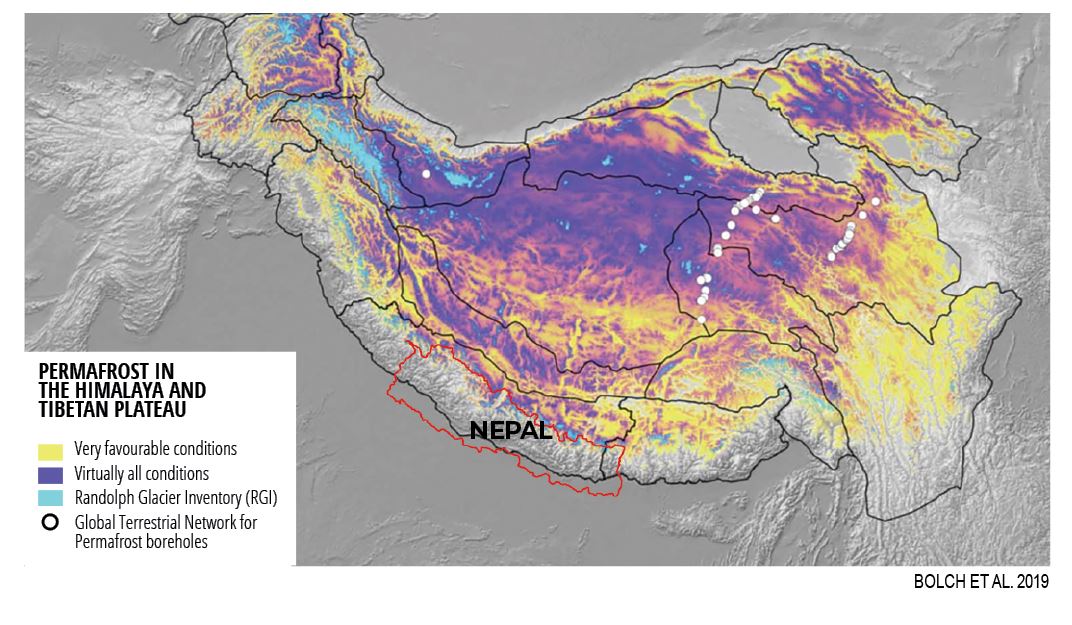
Thawing out
From long-term temperature measurements in deep boreholes, we know that global temperature increase of the atmosphere during the past about 100-150 years has warmed up mountain slopes down to depths below the surface of about 100m. The effect of this deep warming is the reversal of the freezing and cooling effects.
It reduces the strength of rocks or debris with ice and makes them ‘softer’ and more permeable to water. Over the past decades, creep rates of frozen debris in rock glaciers have markedly increased, and the frequency of large rock-ice avalanches from high-mountain permafrost has accelerated.
In the Peruvian Andes, two major events from mountain permafrost occurred in 2020 and 2023. Both had volumes of 1-1.5 million m3, reached lakes at the foot of the slope, and produced flood waves. The 2020 Salkantay flood caused loss of lives and heavy damage at Santa Teresa, 35km down valley. The flood wave at Rasac was fortunately retained within the lake at lower altitude.
On 5 May 2012, a massive rock, ice, and debris avalanche from Annapurna IV cascaded into the Seti River gorge, plummeting into a rockfall-dammed lake. The impact triggered an air blast that levelled thousands of hectares of forests, a dust cloud covered the countryside in fine white rock powder, and a hyper-concentrated slurry flood that killed 72 people downstream.
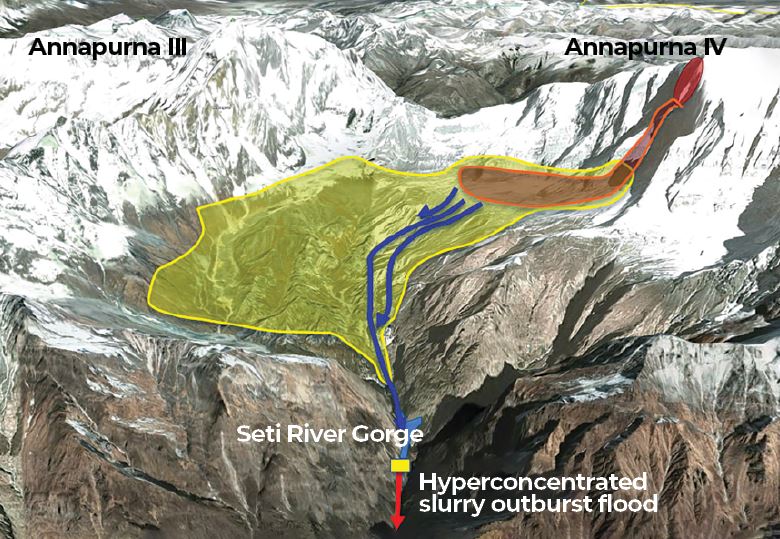
On 20 April 2017, a high altitude breakage of solid rock from the east face of Saldim Peak (6,388m) in the Barun Valley turned into a 1.1 million m3 debris flow of ice and sediment that plummeted 1,200m down to the Langmale Glacial lake below.
Although the lake was less than 0.01km2 in size, the combination of lake water with melting ice from the ice-debris flow triggered a massive hyper-concentrated flood that destroyed forests, pastureland, and trekking lodges for several dozen kilometres to the Barun’s confluence with the Arun.
In India’s Uttarakhand state bordering Nepal, the Chamoli event of 7 February 2021 was catalysed by the detachment of 20 to 30 million m3 of rocks and ice from a frozen, ice-clad peak. The ensuing avalanche developed into a rapidly flowing mass of rocks, ice and water, killing 204 people and heavily damaging an under-construction hydropower infrastructure 35km downstream.
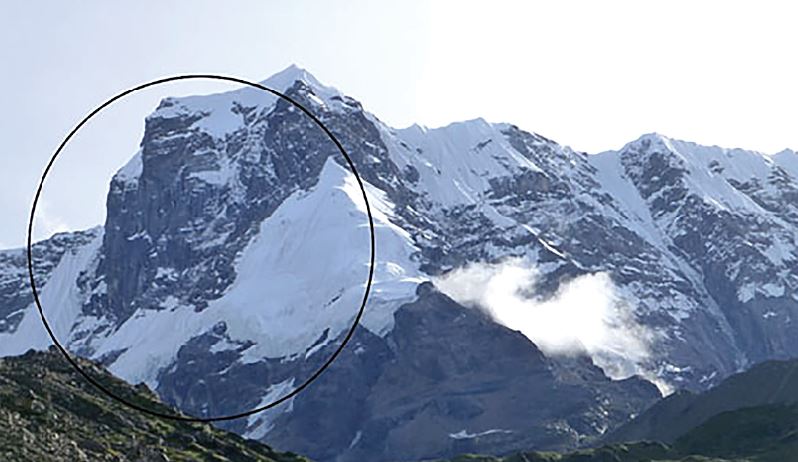
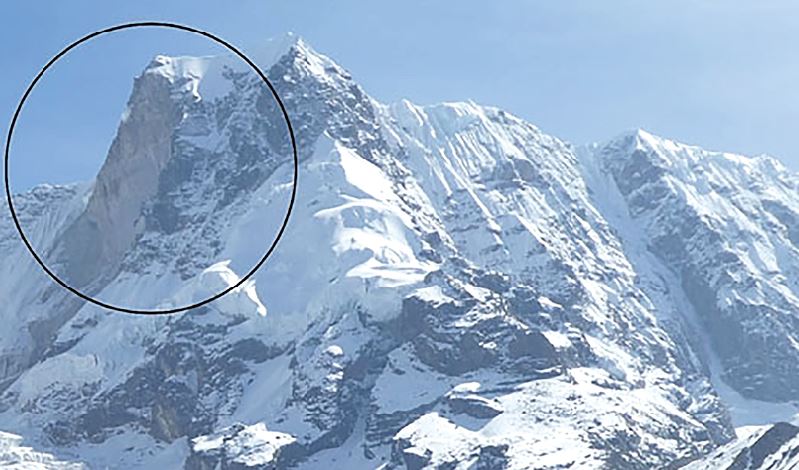
On 3 October 2023, the collapse of about 15 million m3 of debris from a perennially frozen moraine into South Lhonak Lake in the Sikkim Himalaya produced a flood wave and debris flow which killed 14 people with over 100 missing, destroyed a hydropower reservoir at Chungthang, 60km downstream, and caused destruction of houses, roads, and agricultural land.
Similar processes may have been involved in the Birendra Tal glacial lake flood of 21 April 2024 and the Thame flood of 16 August 2024.
Each of the above cases show the potential hazard over long distances related to process chains, especially in connection with impacted mobile water bodies in glacial lakes and artificial reservoirs.
12 TIPS: Awareness and Protective Measures
Changes in high altitude permafrost, and the devastating geomorphic events they cause are still poorly understood by Nepal’s scientific community and government. Earthquakes and heavy rainfall are usually reported by the press to be the primary causes of catastrophic events, both of which are impossible to predict.
However, climate change and gravity, the two major forces at work on Himalayan permafrost, are constants. As permafrost continues to destabilise at the highest altitudes in the Nepal Himalaya, more and more devastating events can be expected to occur.
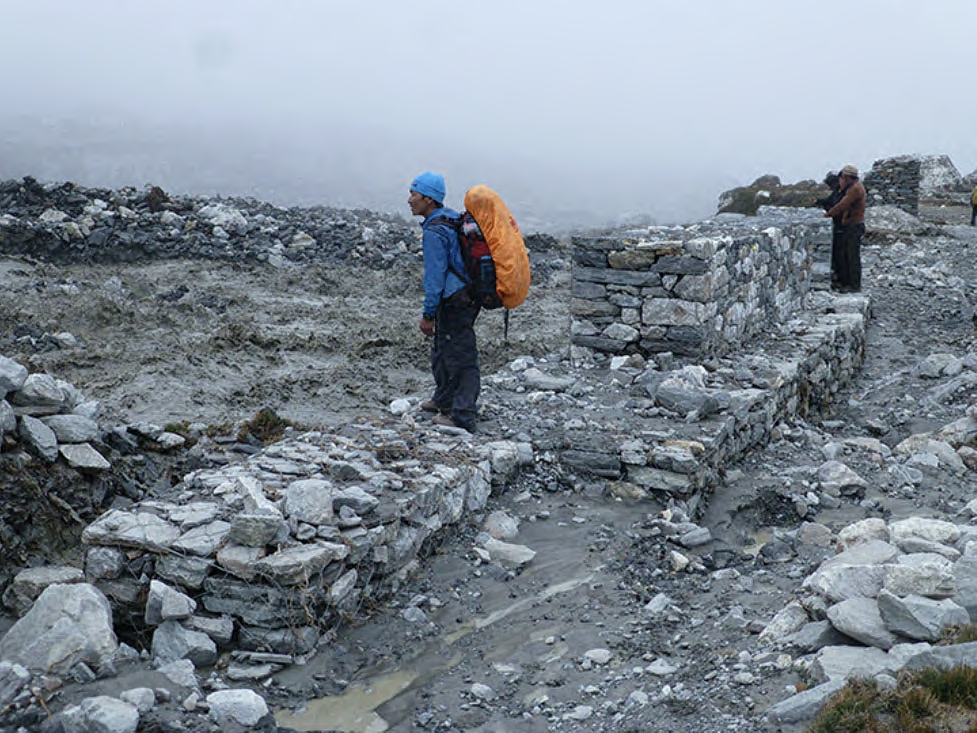
The question is, what can we do about it? Based on our collective experience in the European Alps, Peruvian Andes, and Nepal Himalaya over the past several decades, we offer the following suggestions:
1. Encourage more permafrost and hazard research. Nepal’s scientists and practitioners need to better understand and acknowledge the role of permafrost change in the growing list of climate change-related impacts in the high mountain environment, and more research of an applied nature is indicated. Interdisciplinary approaches that include and involve local people usually yield the most promising and effective results.
2. Standardise glacial lake risk assessment methods. Currently, scientists use various methods to assess a lake’s potential danger of flooding, with predictably mixed and widely differing results. Imja glacial lake in Khumbu, for example, is considered by some scientists to be extremely dangerous, and by others to have a low to moderate risk. The result is confusion among local people, local governments, and scientists alike.
3. Update and revise the current list of potentially dangerous glacial lakes in Nepal. The inventory should incorporate the changing permafrost factor, including smaller lakes less than 0.01km2. Even very small glacial lakes, such as those above Thame and the Langmale glacial lake in the Barun, can be as destructive as large lakes under the right conditions and cascading processes.
4. Develop new feasibility study protocols for large hydropower projects. These guidelines must incorporate the changing permafrost factor as well as the heightened threat of glacier floods. The potentially disastrous impacts of climate change upon major hydropower projects in Nepal have been predicted by scientists for decades, and yet the construction (and destruction) of major, multi-million-dollar installations continues. Hundreds of lives, and millions of dollars of infrastructure, stand to be saved through the use of more detailed, comprehensive evaluations of permafrost and glacier-related risks prior to project implementation.
5. Develop zoning policies. These should discourage the location of farms and infrastructure (homes, tourist lodges) within known and active floodplains. Some scientists believe that most of the deaths from the 2012 Seti flood could have been prevented had settlements not been located directly in the floodplain.
6. Simple is beautiful. Rock-filled wire cages (gabions) can be engineered to effectively divert floods and should continue to be promoted in high mountain regions. In the village of Chukung located directly in the floodplain of the Lhotse Glacier in the Sagarmatha National Park, for instance, the installation of gabions after a glacier flood in 2015 protected the village the following year when a second similar flood from the same glacier occurred.
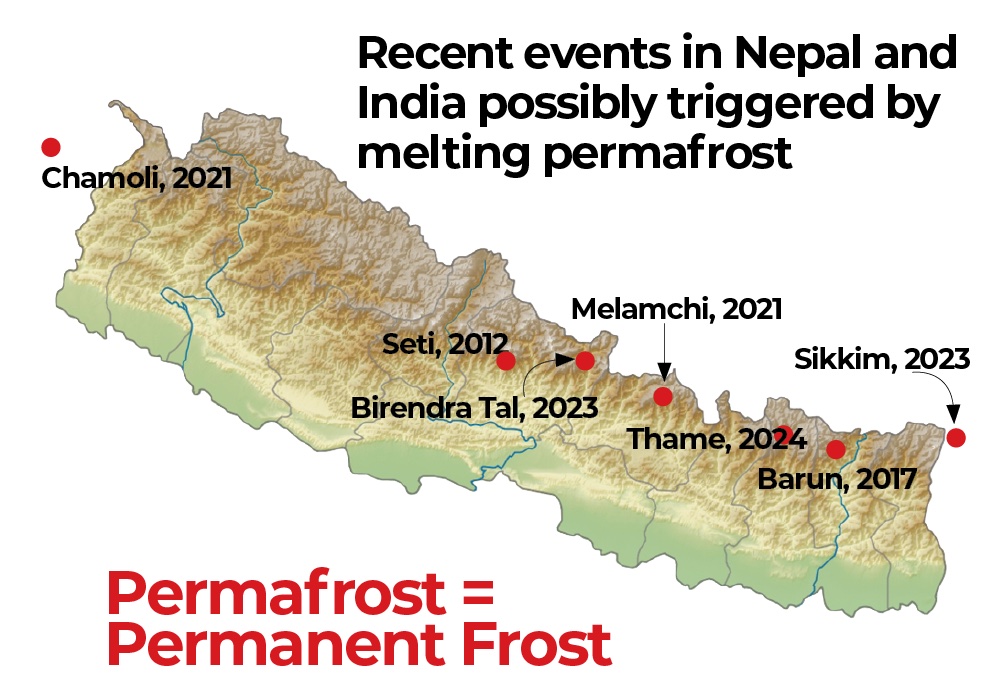
7. Build higher bridges. This can effectively address the growing frequency and magnitudes of glacier floods in the high mountains, eliminating the need for continuous rebuilding.
8. Increase awareness. Nepal’s mountains will continue to destabilise and become even more dangerous in future. Exercising extra caution while crossing a landslide, an active torrent, or a debris-covered glacier is a fact of life in today’s high Himalayan regions. Likewise, constructing a lodge, school, or community building beneath a small and seemingly harmless glacial lake should be discouraged.
9. Promote simple and effective early warning systems. In spite of their much-touted role in disaster mitigation, early warning systems installed to date at potentially dangerous glacial lakes in Nepal have proven to be expensive disappointments. They are typically installed by a remote and distant government agency, little training in their use by local villagers is offered, and local people say they have no idea what the warning sirens sound like nor what they should do if one goes off. Thus far the most effective and proven EWS tool has been the cell phone. Hundreds of lives were saved during the Seti River flood of 2012 when upstream witnesses phoned warnings to their downstream family and friends.
10. Improve mechanisms that allow scientists to communicate. The results of their permafrost and glacier hazard research should directly, quickly, and effectively be communicated to decision-makers. This has proven to be easy to recommend but difficult to implement in most of the world but is nevertheless in need of our constant efforts to achieve.
11. Encouraging transboundary permafrost research. Increased cooperation between scientists and researchers in Nepal, China, and India is needed to help identify potentially vulnerable crossborder rockfall, avalanche, and catastrophic flooding risk.
12. Develop and implement adaptation planning. Continue planning and training programs targeted at permafrost-related floods and other highland-lowland hazards. Local Adaptation Plans for Action (LAPA) have proven to be an effective tool for increasing local awareness of climate impacts as well as planning for mitigation measures.
Dr Wilfried Haeberli is with the University of Zurich and Dr Alton C Byers University of Colorado at Boulder.